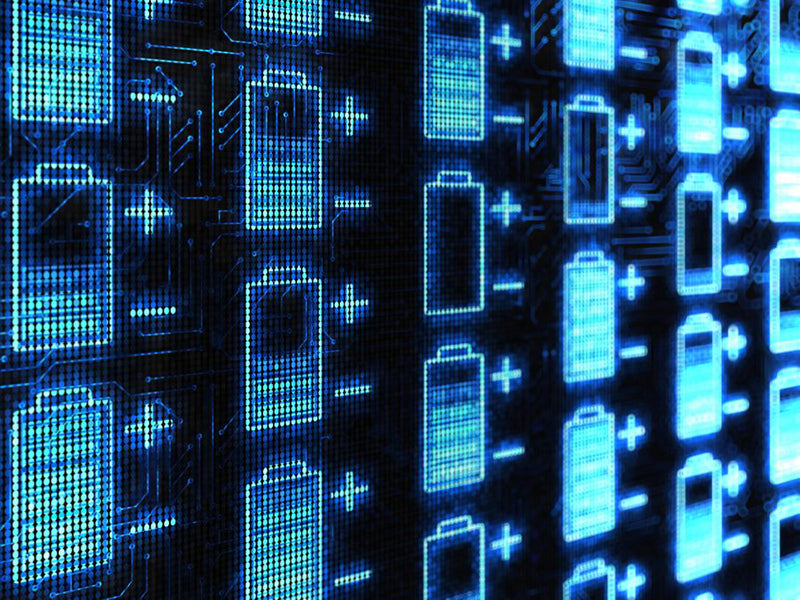
Main content:
1.Focus on the fundamentals of lithium-ion battery
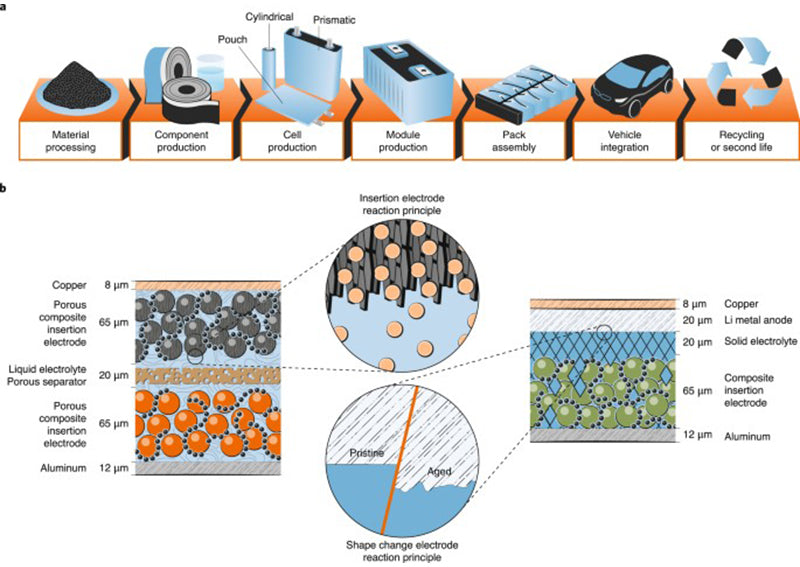
At the current level of development, lithium-ion battery has higher energy density (energy density per unit mass or energy density per unit volume) than other rechargeable electrochemical batteries. The energy density of lithium-ion battery can reach 300 ~ 550W·h/L and 100 ~200W·h/kg, the rated voltage is 3.7V, and the self-discharge rate is low (5%~10% per month), and the operating temperature range is wide. (-20~65℃). The energy densities that can be achieved at the current state of the art are 200W·h/kg and 550W·h/L.
Lithium-ion battery works by using lithium ions in a reversible electrochemical reaction between two materials at different potentials. These two types of active material electrodes are an anode composed of a mixture of oxygen and cobalt (usually LiCoO2 or LiNi0.8Co1.5 Al0.05O2), and a cathode composed of graphite. Sony introduced a new lithium-ion polymer technology in 2008, produced in Singapore, with high energy density (Apelion, 241W·h/kg, 535W·h/L), using lithium cobalt oxide (LiCoO2) and graphite electrodes , using colloidal electrolytes. According to Sony, this lithium-ion battery pushes the limits of lithium-ion technology.
The current research focus of lithium-ion battery is the composition and physicochemical properties of active electrode materials (cathode or anode) to improve the energy density and power density of lithium-ion battery. Of course, in addition to the technical performance requirements, the importance of other aspects to the electrochemical energy storage system is also increasing, including cost, safety, independent production capacity and market potential.
For example, graphite-based electrode materials may generate lithium dendrites during charging. In this case, the safety requirements of users when batteries are grouped are becoming more and more important. It is the preferred solution to use materials with good stability and avoid possible functional failure or even damage.
2.Focus on key players in lithium-ion battery technology
Internationally, Toshiba and Enerdel are researching Li4Ti5O12 anode materials for lithium-ion battery, and Sony is researching anode materials based on tin (Nexelion) doped with some nano-scale metals, such as CoSnC and CoSn nanoparticles. Amorphous composite material (Sn-Co-C) is matched with LiNiMnCo0 anode material, and the fabricated lithium-ion battery allows it to recover to 90% of its capacity within 30min at a charging rate of 2C, and discharge 300 cycles at a rate of 1C. Cycle (capacity is 900mA·h, energy storage is 3.15W·h). Samsung (Sam-sung) SDI is researching Sio-Si materials for lithium-ion battery, and Sanyo (SANYO) is conducting research on depositing silicon metal particles on carbon powder, which is scheduled to be launched in mid-2010. Panasonic is developing rechargeable lithium-ion battery with silicon alloy anodes.
3.Focus on the research and development of lithium-ion battery electrolytes
3.1High Voltage Electrolytes for lithium-ion Battery
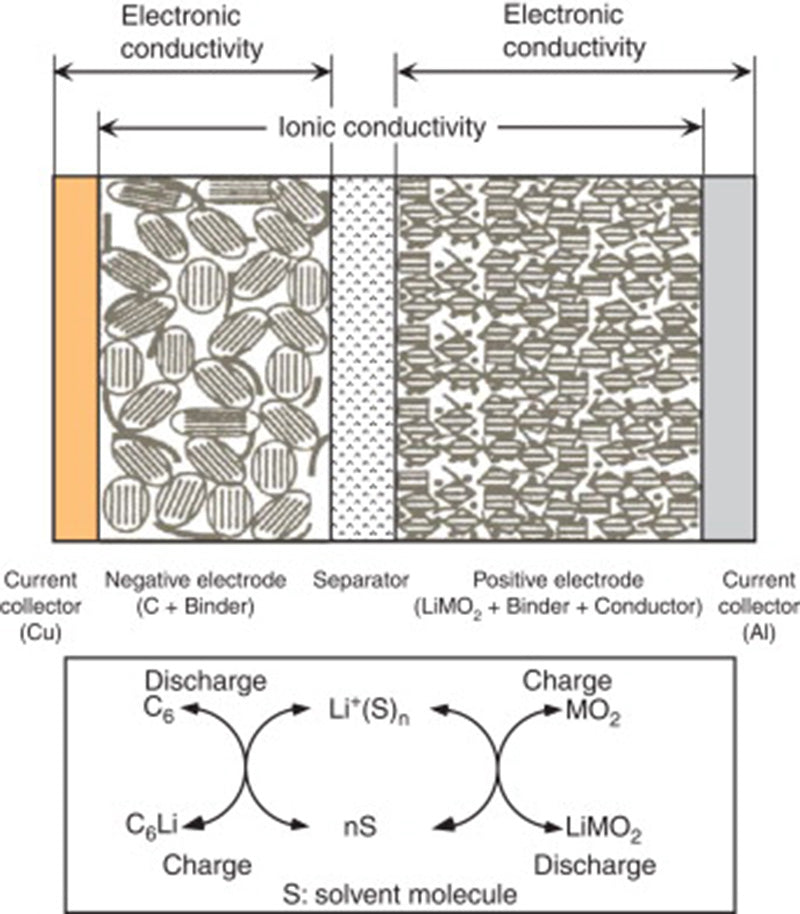
The non-aqueous electrolyte of lithium-ion battery is generally a conductive salt (such as LiPF6) dissolved in a mixed organic carbonate solution (cyclic or acyclic). Its conductivity is significantly lower than that of aqueous electrolytes (about 10 mS/cm, higher than 1 S/cm for sulfuric acid or potassium hydroxide electrolysis). In practical applications, very thin microporous separators (usually less than 20 μm thick) can be used to make up for the insufficient conductivity of non-aqueous electrolytes in lithium-ion battery.
The main areas of research on the above-mentioned lithium-ion battery electrolytes include: ① Enlarging the operating temperature range of the electrolyte from the current 20~60 °C to -40~85 °C; ② Researching non-flammable electrolytes to improve battery safety.
In addition, the electrolyte is also the current limiting factor in the development of high-voltage lithium-ion battery (instability occurs when the voltage exceeds the Li+/Li electromotive force of 4.5V).
Therefore, the development of high-voltage electrolytes is usually limited to the reactivity of the cathode when lithium-ion battery operates at high potentials, especially for Li+/Li potentials higher than 4.5V. Another envisaged solution is to form a Li+ ion conductive film on the electroactive surface of the electrode (active material + conductive agent + current collector) (where Li+ ions are generated from the decomposition or polymerization of additives added in traditional electrolytes), For example, a solid electrolyte interfacial film (SEI) is formed on a graphite electrode.
It can be foreseen that many different types of additives will appear in lithium-ion battery in the future, including polymers (dioxane, biphenyl, etc.), phosphorus compounds [trimethyl phosphite , cerium compounds ( LiBOB sulfur compounds (winds, sultones)], LiF, and liquid ionic compounds, etc.
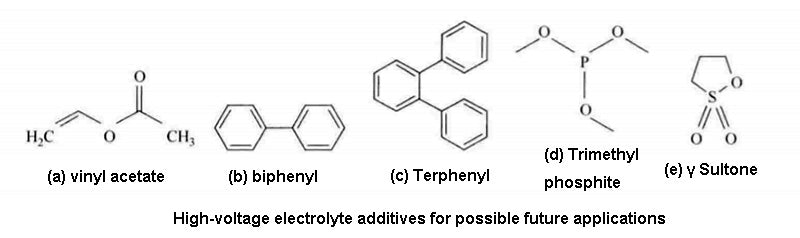
3.2Lithium-ion battery ionic liquid
Due to the high vapor pressure and low ignition point of organic solvents, and the poor thermal stability of LiPF6, this type of electrolyte reduces the safety of lithium-ion battery. The low volatility, non-flammability and good thermal stability of ionic liquids make them potential materials for the preparation of new lithium-ion battery electrolyte systems, which can replace traditional lithium-ion battery electrolytes.
The ionic liquid of the lithium-ion battery is a salt composed of organic cations and inorganic or organic anions in complex combination, and has the characteristics of being in a liquid state at the external ambient temperature. Ionic liquids are non-volatile (will not diffuse in the atmosphere), non-flammable (there is no danger of explosion), high temperature stability (can work at temperatures as high as 200~400 °C depending on the degree of mixing), pro- Aqueous or hydrophobic (according to the characteristics of the anion), viscosity between 37~50cP (at 293K). They are also good electrical conductors (between 0.1 and 15 mS/cm) and have a wide electrochemical window (between 4 and 5 V). Therefore, they can replace volatile organic solvents. Indeed, ionic liquids have some notable advantages, including environmental friendliness (non-volatile, non-flammable), and the ability to modify the properties of positive/negative ion pairs to obtain physicochemical properties tailored to specific application needs. Finally, as inorganic compounds, ionic liquids also possess strong solubility similar to organic solvents.
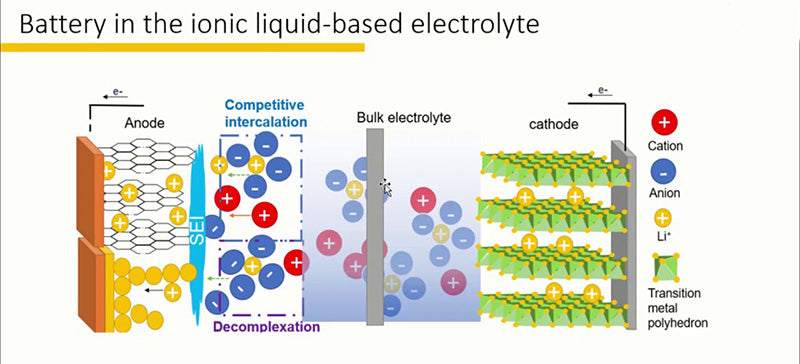
Ionic liquids that can be used as electrolytes for rechargeable lithium-ion battery must have the following characteristics: ① wide electrochemical window; ② weak coordination of anions, thereby reducing the generation of ion clusters in lithium-ion battery (due to the small size of lithium ions. size, this phenomenon of ionic clusters has never completely disappeared in liquids); ③ low viscosity at room temperature, which improves the mobility of ions; ④ wide operating temperature range (such as high thermal stability and high melting point)
Recent studies have shown that although 1,3-imidazolium salts are very good fluid conductors for lithium-ion battery, due to the high cathode potential of 1,3-imidazole anions (about 1V relative to Li+/Li), they are not Suitable for use as an electrolyte for lithium-ion battery. Quaternary (QA) compounds, although very stable electrochemically and easy to synthesize, have until now been rarely used as cations in ionic liquids, and are more difficult to employ than imidazolium salts due to their relatively high melting points. However, when ionic liquids such as pyrrolidine and pyridionine employ cations derived from cyclic quaternary ammonium compounds, they have low melting points and viscosities, as well as significantly higher electrical conductivity than ionic liquids prepared using cyclic cations. Their performance is comparable to that of ionic liquids based on imidazolium cations (eg -18°C for 1-butyl-1-methylpyrrolidine trifluoromethanesulfonyl [TFSI]- melting point). Furthermore, these properties will improve with decreasing cation size.
It has been demonstrated that ionic liquids such as bis(trifluoromethanesulfonyl)imide ([(CF3SO2)2N)-(TFSI]- are composed of relatively small saturated quaternary ammonium cations in combination with electrochemically stable anions. -) gave encouraging results. Its properties include: ① wide electrochemical window, which is due to the low cathodic potential of its saturated quaternary ammonium cation and high anodic potential of [TFSI]- ; ② low viscosity, which is due to the higher flow of [TFSI]- properties and good charge distribution; ③ a wider liquid-phase stability domain, which is determined by the low melting point and high thermal stability of [TFSI]- salts.
Despite these properties, the molar mass and molar volume of the anion [TFSI]- become a clear bottleneck limiting the improvement of electrolyte mobility and ionic conductivity. Therefore, this is a hurdle that needs to be addressed in the application of current [TFSI]- ion electrolyte-based lithium-ion battery. High-power applications cannot be met due to the low conductivity of the electrolyte. In order to improve the performance of ionic liquids based on quaternary amine compounds in lithium-ion battery to meet the application of high energy density systems, it is necessary to develop stable and high-performance anions, such as lower molecular weight and smaller ion size.
In non-aqueous solvent systems, salts such as Li[C2F5BF3] have been found to provide performance comparable to industry standard compounds such as LiPF6 , suggesting that the cathodic and anodic stability of ions such as [RFBF3] is useful in lithium-ion battery The electrolyte is fully competent. Another option is to combine traditional electrolytes with low viscosity with ionic liquids, which are currently of great interest.
Read more: Types and properties of lithium battery