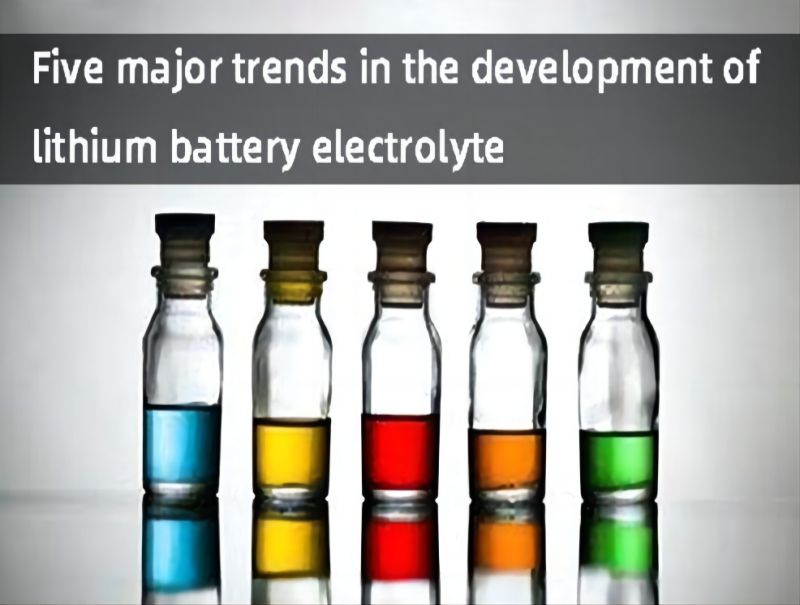
Main content:
Electrolyte is generally composed of electrolyte lithium salt and high purity organic solvent, which is the carrier of lithium ion migration and charge transfer. From the role, electrolyte is the key material that affects the performance of lithium battery such as high voltage and high specific energy. In recent years, with the growing market demand for lithium batteries in new energy vehicles, energy storage and other applications, the production of electrolyte in China is also increasing.
However, at the same time, the continuous development and iteration of new technologies and processes for lithium batteries also put forward higher requirements for the upgrade applicability of electrolyte in terms of high nickel, high voltage, high specific energy and high safety.
For example, high nickel cathode material has become the mainstream technology route for current high energy density lithium batteries. With the increasing proportion of nickel and the use of silicon carbon cathode, new challenges are brought to the R&D and production of electrolyte.
High voltage
In recent years, new high voltage cathode materials such as lithium nickel manganate and layered lithium-rich manganese based materials have been gradually developed, and their discharge voltage can reach more than 5V, but they are still not commercially applied. The most important reason is that the working voltage of current commercial electrolytes cannot be matched.
The current research on electrolytes mainly uses LiPF6 as lithium salt and single or mixed carbonate solvents as main solvents, mainly including EC, DMC, EMC, DEC and PC. when the operating voltage > 4.3 V, the conventional electrolytes usually decompose, which is due to the common organic carbonate solvents, such as chain carbonate DMC, EMC, DEC, and ring carbonate PC, EC, etc. are unstable at high voltages.
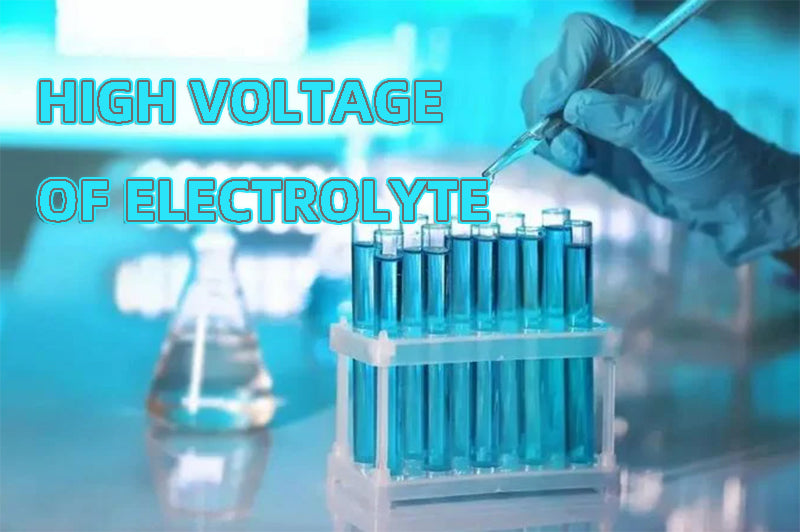
Currently, more research is conducted to increase the working voltage of electrolyte by using high voltage materials, including the use of high voltage solvents, high voltage additives and high concentration lithium salt electrolytes.
High-voltage solvents mainly include fluorine substitute solvents, sulfone solvents, nitrile solvents, ionic liquids, etc. Compared with high-pressure solvents, high-pressure additives are more popular because of the low dosage, obvious effect and low cost, and at present, there are mainly boron additives, benzene derivatives and heterocyclic additives, phosphite additives and so on.
In addition, ether, sulfur, silicone, ionic liquids and other additives are also widely studied and applied. High-concentration lithium salt electrolytes are electrolytes with lithium salt concentration above 3 mol/L. These electrolytes have excellent oxidation/reduction resistance, can prevent corrosion of aluminum collectors, and have high multiplication performance of the battery.
The lithium salt with the highest degree of commercial application is LiPF6, which is prone to decomposition and HF generation in the presence of trace water or at high temperature, destroying the electrode structure and unable to meet the requirements of high voltage and high safety electrolytes, and needs to be modified, while new lithium salts such as lithium bis(fluoroamidomethyl)imide (LiFSI) and lithium bis(trifluoromethanesulfonyl)imide (LiTFSI) are of great interest due to their good thermal stability and insensitivity to moisture.
High power
For power batteries, achieving fast charging is also an important direction for electrolyte development. Li-ion battery high power electrolyte is mainly studied in 2 aspects of performance: first, the SEI membrane charge migration impedance increases under high rate charging, which increases the electrode polarization during charging; again, under high rate charging conditions, Li-ion battery is prone to lithium precipitation in the late stage of constant current charging, which leads to deterioration of SEI membrane condition and deterioration of battery performance.
Therefore, firstly, the high power performance of the battery can be improved to a certain extent by adding lithium salts optimized for high rate charging and discharging. Secondly, the high power performance of the battery can be improved by adding high power performance additives, reducing the charge transfer impedance of the electrode interface under high rate charging and discharging by adding film forming additives with better effect than EC, or adding lithium salt deposition improvers to prevent the growth of lithium branched crystals during high rate charging.

Wide temperature range
Multiple application scenarios reflect both the advantages of lithium batteries and the driving force for their development. In application scenarios such as electric vehicles and portable electronic devices, lithium batteries only need to meet the operating temperature of 15~35°C. However, in some special application scenarios, lithium batteries are required to operate at 15~35°C. However, in some special application scenarios, lithium batteries are required to break through this temperature range.
Too low operating temperature slows down the kinetic process of electrochemical reactions within Li-ion batteries. Due to the increase in electrolyte viscosity, decrease in electrolyte salt solubility, and increase in Li+ desolvation energy barrier, the rate of charge transfer from ion diffusion within the electrolyte and electrode material to the electrode-electrolyte interface is significantly reduced at each stage of charge transfer at low temperatures, which can cause increased cell polarization, decreased capacity, and even difficulty in operation.
In addition, too low operating temperature may change the electrochemical reaction path, such as at low temperature, Li+ which should be embedded in the graphite cathode layer may be reduced on the graphite cathode surface, forming dendrites and endangering the safety of the battery. And in high temperature operating environment, the main challenge of Li battery comes from excessive side reactions because the stability of electrolyte/electrode interface decreases and the electrode and electrolyte side reactions cannot be prevented.
Moreover, too high operating temperatures are challenging for the thermal stability of electrode materials. A considerable amount of research has been reported on electrolyte materials for lithium batteries with a wide operating temperature range.
Despite the advantages of solid electrolytes at high temperatures, the use of solid electrolytes requires a revolution of the existing battery production system and brings additional costs, so a more feasible solution at this stage is to optimize the composition of liquid electrolytes as a solution to the problems faced by high and low temperature batteries.
At present, the optimization of wide temperature electrolyte mainly focuses on improving the boiling point and flash point of electrolyte, reducing the solidification point and viscosity of electrolyte, and improving the stability of electrolyte/electrode interface.

Intelligent safety
When the local temperature of lithium battery system increases, the reaction inside the battery goes out of control and further leads to internal short circuit due to the thermal contraction of the diaphragm, releasing more heat and leading to complete thermal runaway of the final lithium battery. The mechanism of action of thermally responsive polymer electrolytes is mostly based on the principle of phase change, i.e., reversible phase change from hydrophobic to hydrophilic.
The polymer molecular conformation changes reversibly when the temperature increases beyond a critical value. This conformational change leads to a decrease in the ionic conductivity of the polymer itself and an increase in the charge transfer impedance of the lithium battery, thus preventing the occurrence of thermal runaway. When the temperature decreases, the thermosensitive polymer can be redissolved in the electrolyte to restore its ionic conductivity.
Among ionic liquids, thermally responsive polymers such as polyethylene oxide (PEO), polybenzyl methacrylate (PBMA) and polyn-butyl methacrylate all have low critical solution temperatures between 80 and 200°C, which completely cover the thermal runaway temperature of lithium batteries and can be used in lithium batteries.
Long cycle
Since there are still major technical difficulties in the recycling of lithium batteries, especially power batteries, improving the cycle life of batteries is a way to alleviate this situation. Scientists have proposed a self-purifying electrolyte, 1.6M lithium bis(fluorosulfonyl)imide salt (LiFSI) dissolved in a functional nitrile solvent 3-(triethoxysilyl)propanenitrile (TEOSCN), TEOSCN in the electrolyte can effectively remove the active harmful substances in the electrolyte, where FSI-although at a lower concentration can still form a lower interfacial impedance in graphite and Ni-rich cathode.
This self-purifying electrolyte achieves long and stable cycles of MCMB||NMC811 full cells, with 91% capacity retention after 1000 cycles at 25°C and 81% capacity retention after 500 cycles at 60°C. Even after exposing the electrolyte to air for 1 hour, the electrolyte can still maintain stable cycling of the MCMB||NMC811 full cell, which greatly simplifies the production process and lithium ion battery prices.
Related article: lithium ion battery electrolyte, top 5 battery electrolyte companies, lithium ion battery life