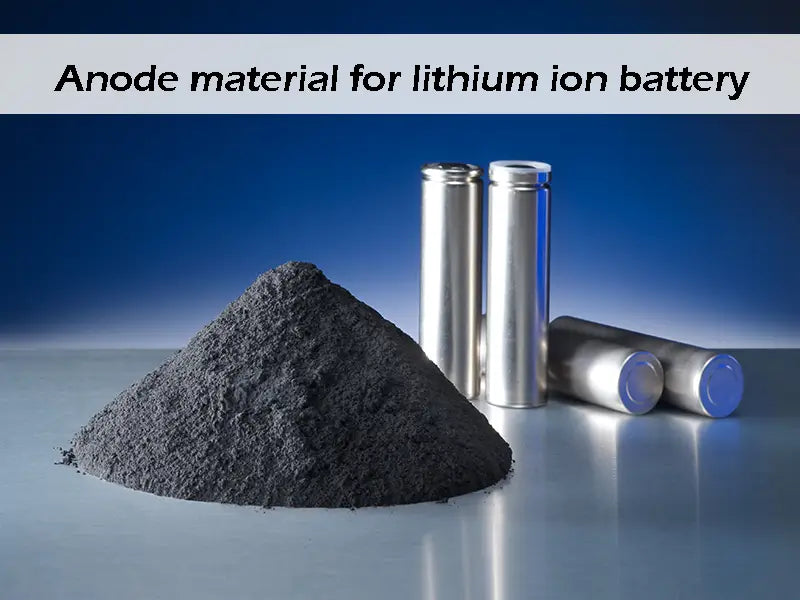
Main content:
The key to the successful application of ion batteries lies in the preparation of anode materials that can reversibly intercalate and deintercalate lithium ions. Such materials are required to have: ① small change in free energy during the intercalation reaction of lithium ions; ② high diffusivity of lithium ions in the solid-state structure of the anode; ③ highly reversible intercalation reaction; ④ good electrical conductivity; ⑤ thermodynamics It is stable and does not react with electrolytes at the same time. At present, research work mainly focuses on carbon materials and other compounds with special structures.
1. Carbon anode material
Carbon anode lithium-ion batteries show good performance in terms of safety and cycle life, and carbon materials are cheap and non-toxic. At present, commercial lithium-ion batteries widely use carbon anode materials.
As we all know, there are many kinds of carbon materials. At present, the more and more successful carbon anode materials are graphite, acetylene black, microbead carbon, petroleum coke, carbon fiber, cracked polymer and cracked carbon. Among the many materials used as carbon anodes, natural graphite has low intercalation potential and excellent intercalation-deintercalation performance, and is a good anode material for lithium ion batteries. Usually, the theoretical expression of the compound formed by lithium in carbon materials is LiC6, and the theoretical specific capacity according to the stoichiometry is 372 mA·h/g. In recent years, with the deepening of the research on carbon materials, it has been found that through the surface modification and structural adjustment of graphite and various carbon materials, the graphite is partially disordered, or the nano-scale is formed in various carbon materials. The structure of pores, holes and channels, in which the intercalation and deintercalation of lithium can not only be carried out according to stoichiometric LiC6, but also non-stoichiometric intercalation and deintercalation, and its specific capacity is greatly increased. The theoretical value of LiC6 is 372mA· The h/g is increased to 700~1 000mA·h/g, so the specific energy of the lithium-ion battery is greatly increased. In recent years, the research work of lithium-ion batteries has focused on the research of carbon anode materials, and many new progress has been made. Okuno et al. studied the coke electrode modified with mesophase pitch coke and found that the specific capacity of the coke electrode was only 170~250 mA h/g. The coke and MPC were mixed in a ratio of 4:1, and the specific capacity was 277 mA h/g. The specific capacity of the coke electrode modified with MPC is 300~310 mA·h/g. Ma Shuhua et al. artificially deposited a layer of Li2CO3 or LiOH film on the mesophase microsphere graphite electrode, and the capacity of the anode and the first charge-discharge efficiency were improved to a certain extent. The natural gas coke prepared by Deng Zhenghua et al. using thermionic pyrolysis of natural gas has good Li intercalation capacity, the initial discharge capacity is 402 mA h/g, the charging capacity is 235 mA h/g, and the charge-discharge efficiency is 58.5%. Feng Xikang et al. treated petroleum coke at 2600 ℃ in a reducing atmosphere and obtained an external coating of artificial graphite with a carbon layer. Low self-discharge rate.
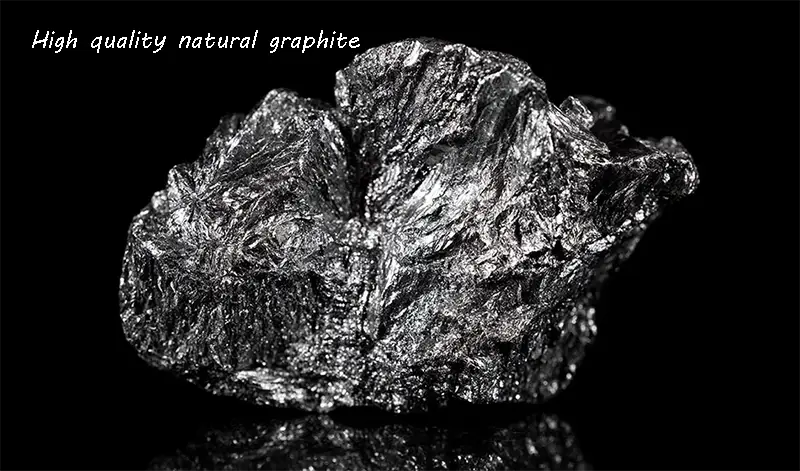
Sanyo uses high-quality natural graphite as the anode. The graphite reacts with an appropriate amount of water vapor at high temperature to make the surface amorphous, so that Li+ can be easily embedded in the graphite lattice, thereby improving its ability to intercalate Li. The Li-intercalation ability of carbon anode is different for different materials, which is mainly affected by its structure. For example, Sony uses polyfurfuryl alcohol compounds, Sanyo uses natural graphite, and Panasonic uses mesophase pitch-based carbon microspheres. Generally speaking, amorphous carbon has large interlayer spacing and small layer plane, such as graphite is 0.335nm, coke is 0.34~0.35nm. Some hard carbons are as high as 0.38nm, and the diffusion rate of Li ions in it is faster. , which can charge and discharge the battery faster. Dohn described the relationship between the graphite interlayer spacing d002 and the specific capacity, indicating that the discharge specific capacity increases with the increase of d002. Takami studied the interlayer spacing and diffusion coefficient of mesophase pitch-based fibers at different temperatures, and believed that the interlayer spacing depends on the degree of graphitization of carbon, and the increase of the degree of graphitization can reduce the activation energy of Li ion diffusion, and is conducive to the diffusion of Li ions .
Carbon anode materials with high specific capacity can greatly improve the specific energy of lithium-ion batteries, but partially cracked carbides have an obvious defect that is voltage hysteresis, that is, Li ions are embedded at around 0V during charging, and desorbed at 1V during discharge. Embedded, although the charging voltage of such batteries is 4V, but the actual working voltage is only 3V. Takami et al believe that phenolic resin, polyaniline, microbead carbon, etc. have obvious voltage hysteresis. In addition, the preparation process of such anode materials is complicated and the cost is high.
The disadvantage of using natural flake graphite as a anode material for lithium ion batteries is that the graphite layers are bound by weak intermolecular forces, namely van der Waals forces. Residues are generated and a new surface is formed, and the organic electrolyte is continuously reduced and decomposed on the newly formed surface to form a new SEI film, which not only consumes a large amount of lithium ions, but also increases the loss of the first irreversible capacity. Intercalation and deintercalation can cause volume expansion and contraction of graphite particles, resulting in partial interruption of the energizing network between particles and poor cycle life.
It is also reported that in early 2008, Mitsui Metal Mining successfully developed a new cathode called "SILX", which uses thin metal copper to cover silicon material and a physical structure that allows expansion. It is reported that the capacity of lithium batteries can be increased by about 50% , the life of the anode is the same as that of the existing lithium battery.
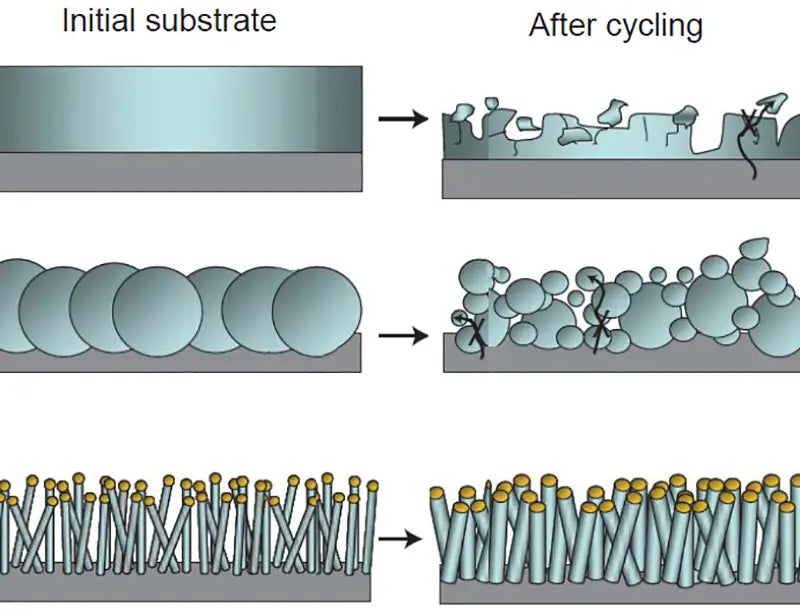
2. Non-carbon anode materials
In recent years, the research on non-carbon anode materials for LIB is also very extensive, which can be generally divided into lithium transition metal hydrides, transition metal oxides and nano-alloy materials according to their composition. Lithium transition metal nitrides have good ionic conductivity, electronic conductivity and chemical stability, and are used as anode materials for lithium ion batteries, and their discharge voltage is usually above 1.0V. The discharge specific capacity, cycle performance and smoothness of charge and discharge curves of electrodes vary greatly depending on the type of material. For example, when Li3FeN2 is used as the anode of LIB, the discharge capacity is 150mA·h/g, the discharge potential is around 1.3V, the charge and discharge curves are very flat, and there is no discharge hysteresis, but the capacity has a significant attenuation. Li3-x CoxN has a high discharge capacity of 900 mA h/g, and the discharge potential is around 1.0V, but the charge and discharge curves are not stable, with obvious potential hysteresis and capacity decay. At present, in order to achieve practical application of such materials, further in-depth research is needed. SnO/SnO2 used as LIB anode has the advantages of high specific capacity and relatively low discharge potential, but its first irreversible capacity loss is large, the capacity decays rapidly, and the discharge potential curve is not stable. The electrochemical properties of SnO/SnO2 are quite different due to different preparation methods. For example, the reversible capacity of SnO2 prepared by low-pressure chemical vapor deposition method is more than 500mA·h/g, and the cycle life is relatively ideal, and there is no decay after 100 cycles. Introducing some non-metal and metal oxides, such as B, Al, Ge, Ti, Mn, Fe, etc. into SnO and heat treatment, amorphous composite oxides can be obtained, which are called amorphous tin-based composite oxides and Compared with tin oxides, the cycle life of tin-based composite oxides has been greatly improved, but it is still difficult to achieve industrialization standards.
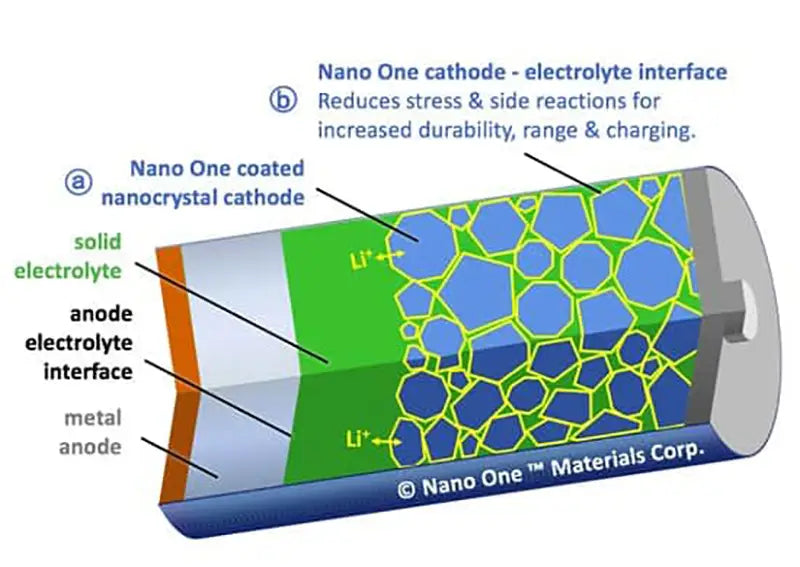
Nano anode materials mainly hope to use the nano-features of materials to reduce the influence of volume expansion and contraction on the structure during charging and discharging, thereby improving the cycle performance. Practical applications show that the effective utilization of nano-features can improve the cycling performance of these anode materials, however, it is still some distance away from practical applications. The key reason is that the nanoparticles gradually combine with the progress of the cycle, thus losing the unique properties of the nanoparticles, resulting in the destruction of the structure and the attenuation of the reversible capacity. In addition, the high cost of nanomaterials has also become a major obstacle limiting their applications.
When certain metals, such as Sn, Si, Al, etc., intercalate lithium, they will form lithium-metal alloys with high lithium content. For example, the theoretical capacity of Sn is 990mA·h/cm3, which is close to 10 times the theoretical volume specific capacity of graphite. The main problems of alloy anode materials are low initial efficiency and cycle stability, and the electrode structure damage caused by the volume effect of the anode material during repeated charge and discharge must be solved. Simple metal anodes have poor cycle performance and poor safety. The use of alloy anodes combined with other flexible materials is expected to solve these problems.
In short, non-carbon anode materials have high volumetric energy density, which has attracted more and more interest of scientific researchers, but there are also shortcomings such as poor cycle stability, large irreversible capacity, and high material preparation costs, which have not been realized so far. industrialization. The development trend of anode materials is to improve the capacity and cycle stability as the goal, and to research and develop new applicable high-capacity, carbon composite anode materials by compounding carbon materials with various high-capacity non-carbon anode materials through various methods.
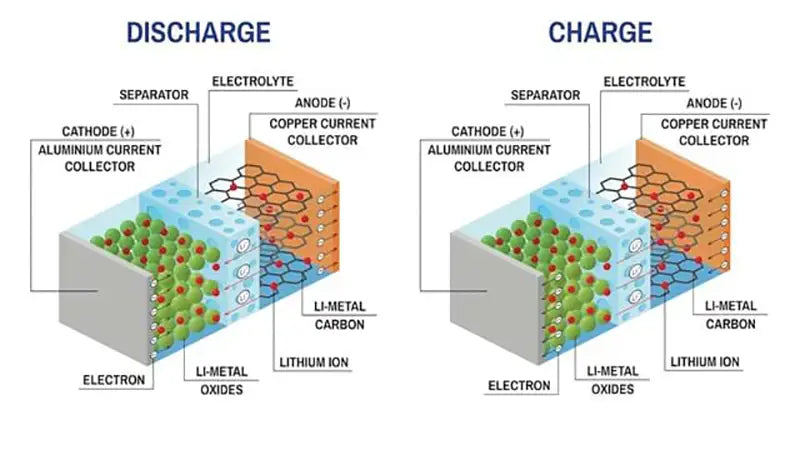
The US Department of Energy's Argonne National Laboratory signed a technology transfer contract with BASF on June 6, 2009. BASF will use Argonne National Laboratory's patented composite anode material to produce advanced lithium-ion batteries. BASF will build North America's largest anode material production facility in Elyria, Ohio, and will further develop applications for cathode and anode materials for lithium-ion batteries at its Beachwood, Ohio, U.S. plant.